Circumstellar
Disks with Planets
Introduction
Over the past few years, considerable
efforts have been made to examine the gravitational perturbations induced
in a circumstellar disk by an orbiting planetary mass body. Gravitational
interactions between the planet and the surrounding material lead to an exchange
of angular momentum. As a result, both the morphology of the disk and the
orbital elements of the planet are affected.
The most prominent features arising in a disk containing a planet are a low-density
annular region along the planet's path (known as gap) and spiral wave
patterns, emanating from the Lagrangian points. The extent to which the disk
is marked by such signatures depends on the mass of the perturbing body
as well as on the viscosity of the circumstellar medium.
The importance of the peculiar appearance of circumstellar disks hosting planets
lies in the possibility that it may be exploited for identifying and observing
planets still in the formation process.
The results presented here have been obtained by performing two-dimensional
thermo-hydrodynamical
simulations of circumstellar disks with embedded planets of different masses.
Furthermore, various viscosity regimes have been considered. In order to
investigate separately what kind of bearing these physical properties has
on the disk morphology, two comparisons are shown:
- Disks with different viscosities
- Planets with different masses
Disks with Different Viscosities
Simulations of a Jupiter mass (M J)
protoplanet embedded in disk models with kinematic viscosity ν=10 15,
5×10 15, and 10 16 cm 2 s -1
have been carried out. Viscosity acts to smooth out all dishomogeneities,
and therefore it tends to suppress the formation of the gap. The main outcomes
of this comparison are:
- Only when the viscosity is small enough a deep and
wide gap is carved in by the planet, otherwise it reduces to a shallow
trough.
- The gap feature is a property affecting not only
the density distribution but also the distribution of pressure, temperature,
optical thickness, and radiated flux.
- Average temperatures inside the gap can be rather
cold and even lower than 20 K.
- The relative disk thickness H/r is on the order of
0.04 and its shape is such to shade the planet against the stellar radiation.
Some of these points are illustrated in the images and the graphs presented
below.
|
Surface Density |
Midplane Temperature |
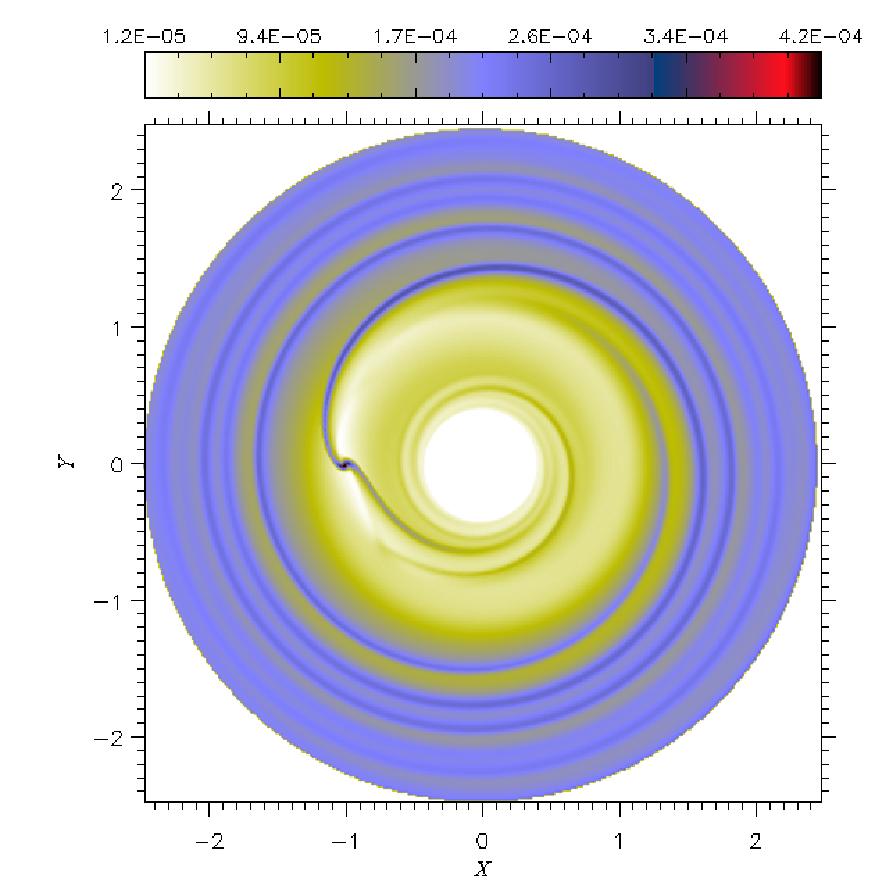 |
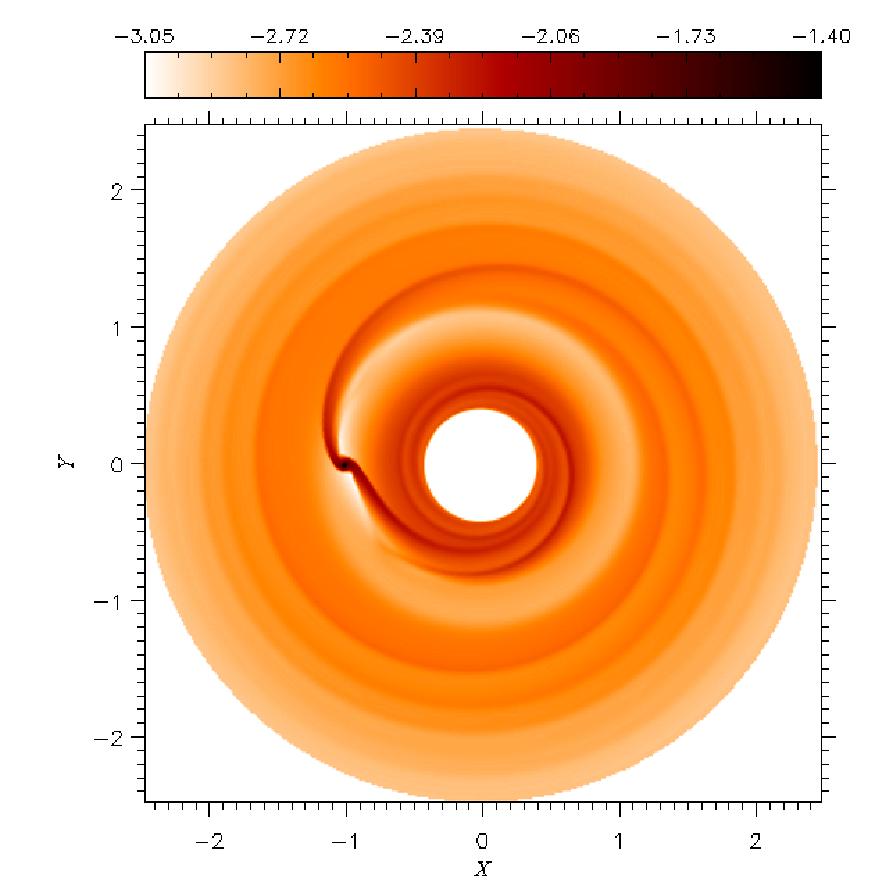 |
The images above show
the surface density (left) and the midplane temperature (right)
of a disk with ν=1016 cm2 s-1. The images
below refer to the same quantities within a disk with ν=1015cm2
s-1. Temperature distribution is in log-scale. The planet is
located at (X,Y)=(-1,0). In color-bar units, 10-4corresponds to
33 g cm-2 and 10-2 equals 198 K. [Click on the images
to enlarge]. |
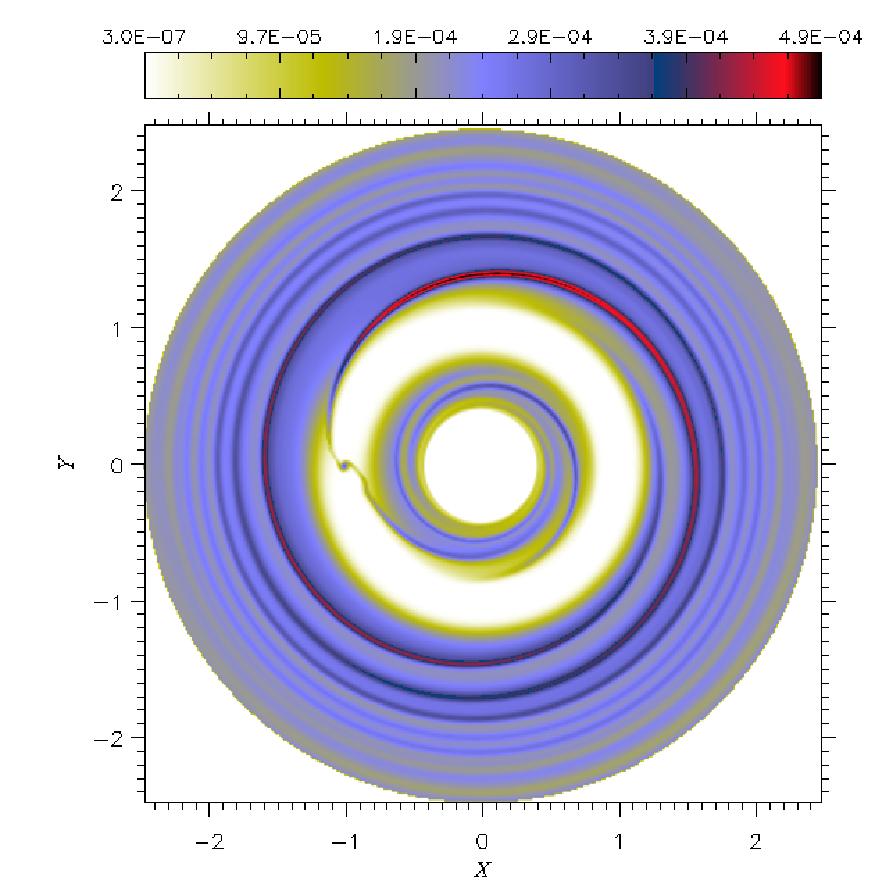 |
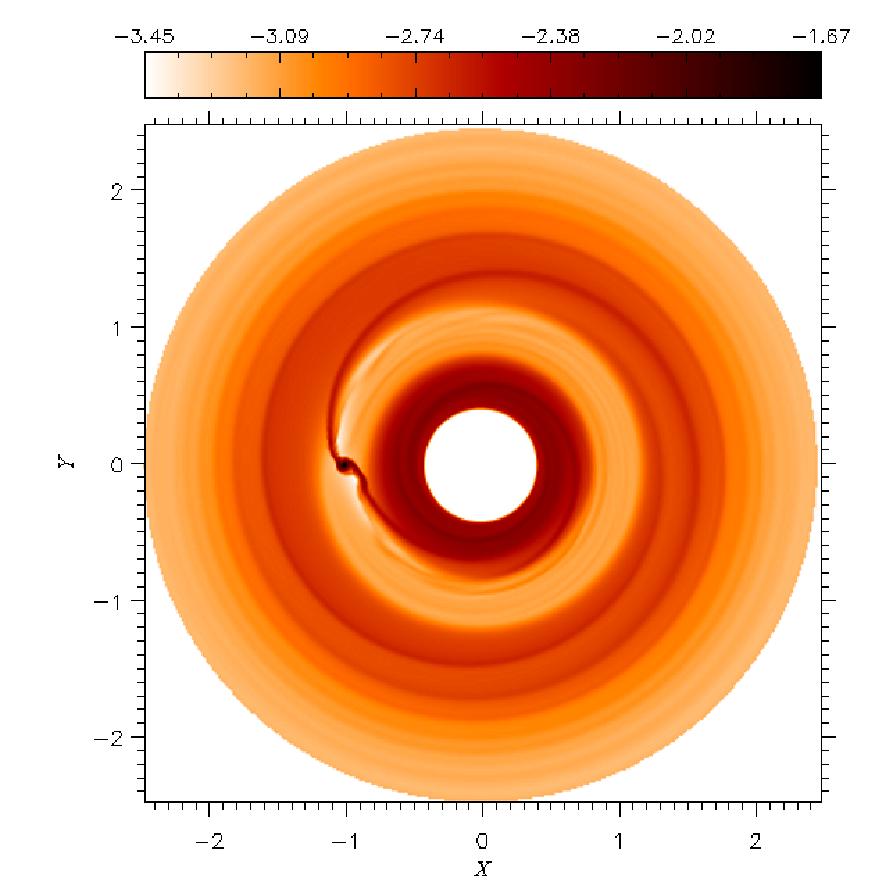 |
|
Average Surface Density
|
Average Midplane Temperature
|
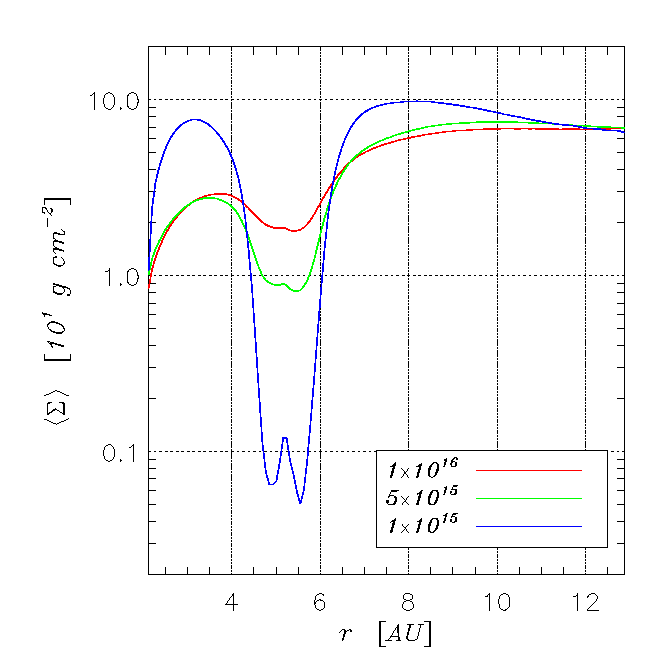 |
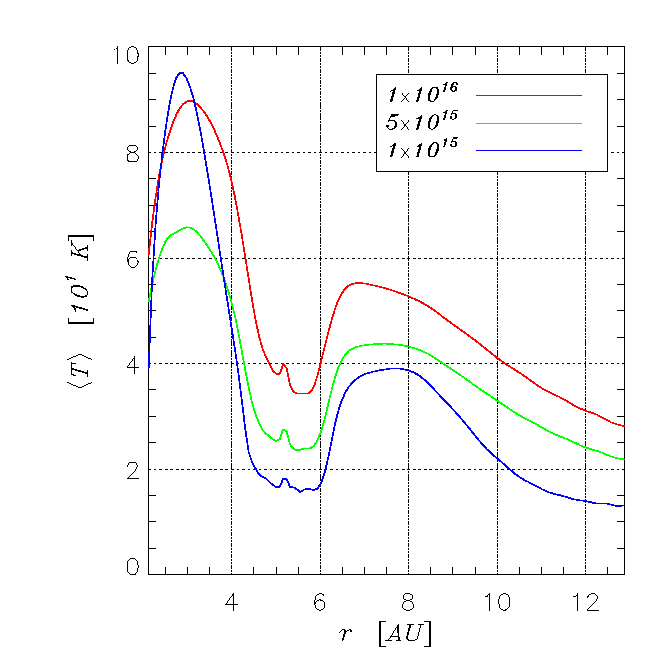 |
The above plots show
the azimuthally averaged surface density (left) and the midplane temperature
(right) of disks with different kinematic viscosities (values quoted
in the legend are in cgs units). As viscosity becomes larger, viscous torques
grow stronger and more efficient in opposing gravitational torques. Hence,
the gap is partially filled in. |
|
Average Disk Thickness |
|
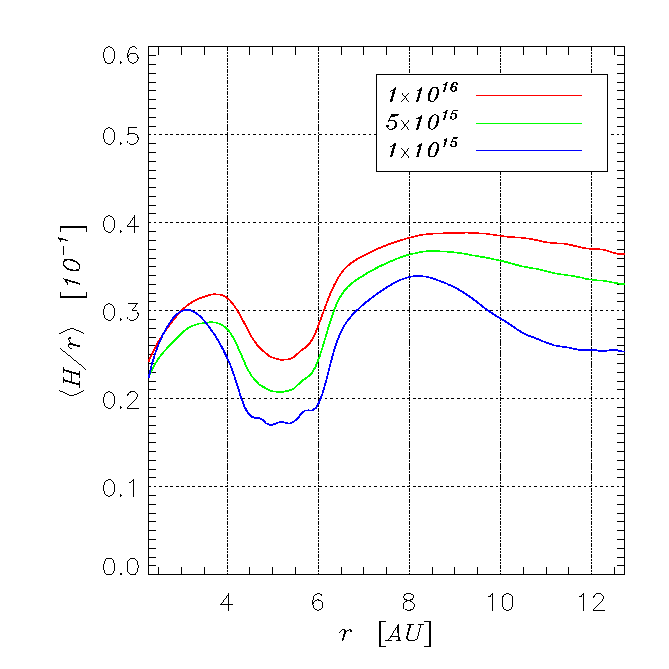 |
The plot indicates how the azimuthally
averaged aspect ratio of a disk (i.e., H/r) varies according to the magnitude of the kinematic
viscosity (see legend). The trough at the planet location is generated by
the temperature gap combined with the gravitational field of the planet. As
a result of this disk thickness profile, the planet can be shaded against direct
radiation from the central star. [Click on the image to enlarge].
|
|
Emitted Flux
|
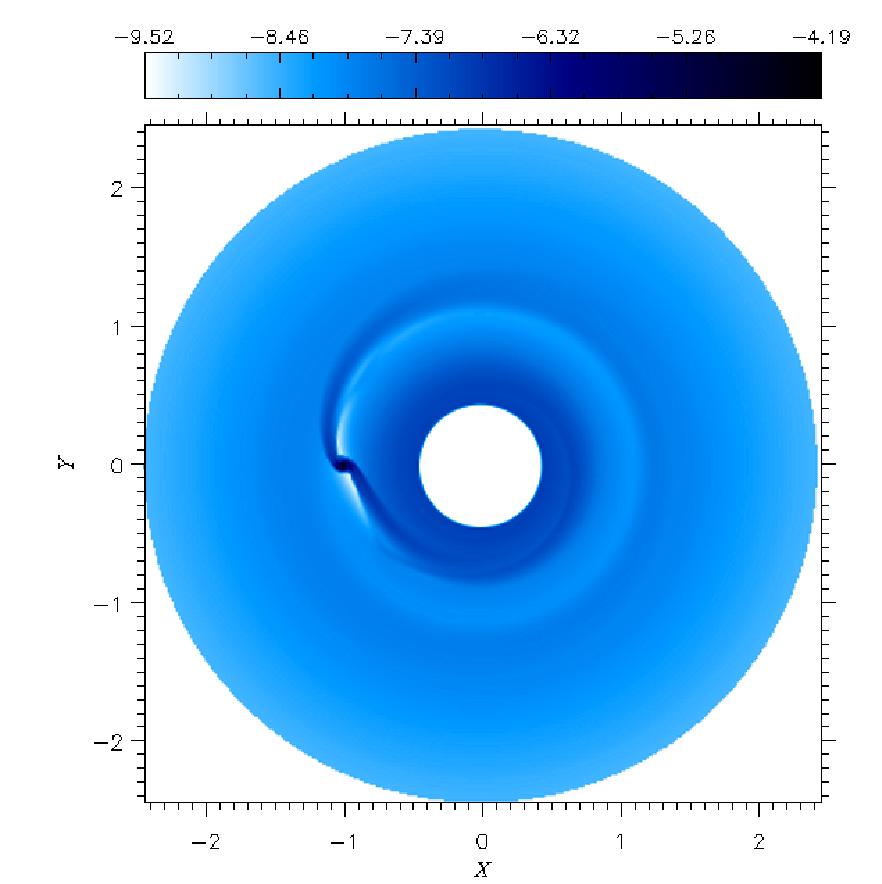 |
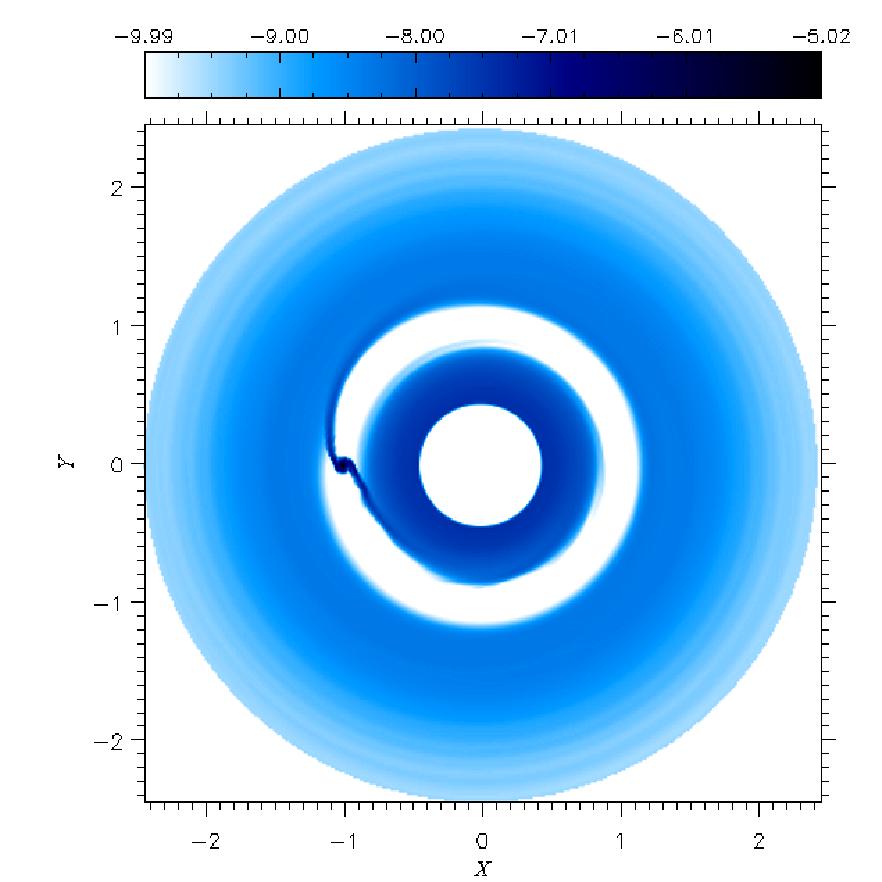 |
This above are the
fluxes emitted by a disk with viscosity ν=1016 cm2
s-1 (left) and with ν=1015 cm2 s-1
(right). In the plot units, 10-5 corresponds to 4.7×104
erg cm-2 s-1, which is six orders of magnitude smaller
than the surface flux of the Sun. [Click on the images to enlarge].
|
Planets with Different Masses
Along with the investigation of effects
due to viscosity, the impact of the planetary mass on the disk morphology
has been also examined by performing computations of 0.1, 0.2, and 0.5 MJ
objects. The ability of a planet to dig a gap greatly reduces when its mass
is smaller than roughly 0.2 MJ (66 Earth masses), especially
if it dwells in a high viscosity environment. This is quantitatively shown
below.
|
|
|